Dynamic Behavior of Sand with NanoParticles Comportement dynamique de Sable ave
Dynamic Behavior of Sand with NanoParticles Comportement dynamique de Sable avec Nanoparticules Felipe Ochoa-Cornejo Civil Engineering Department, University of Chile, Chile, fochoa@ing.uchile.cl ABSTRACT: This work presents an experimental investigation to examine the effect of Laponite -a synthetic nanoclay with high plasticity- on the dynamic behavior of sands. The experimental program considers cyclic triaxial and resonant column tests on specimens of pluviated sand and Laponite. Microstructure observation address the granular fabric and the sand-Laponite interactions. Cyclic triaxial tests show that 1% of Laponite impacts the cyclic behavior, improving the liquefaction resistance over one order of magnitude compared to clean sands. Resonant column tests show that the small strain stiffness of the sand (G0) increases with 1% of Laponite. The overall results show that Laponite extends the linear threshold of deformation, reduces stiffness degradation, and delays excess pore pressures. Microstructure observations suggest that Laponite reduces sand particle mobility by the formation of a pore fluid with solid-like properties. RÉSUMÉ: Ce travail présente une étude expérimentale pour examiner l'effet de la Laponite -une nanoargille synthétique avec une plasticité élevée- sur le comportement dynamique des sables. Le programme expérimental envisage des essais triaxiaux cycliques et de colonne résonnante sur des échantillons de sable pluvié et de Laponite. L'observation de la microstructure concerne le tissu granulaire et les interactions sable-Laponite. Les essais triaxiaux cycliques montrent que 1% de Laponite affecte le comportement cyclique, améliorant la résistance à la liquéfaction sur un ordre de grandeur par rapport aux sables propres. Les essais de colonnes résonantes montrent que la rigidité à faible déformation du sable (G0) augmente de plus de 20% avec 1% de Laponite. Les résultats globaux montrent que la Laponite prolonge le seuil linéaire de déformation, réduit la dégradation de la rigidité et retarde les pressions de pores en excès. Les observations de microstructures suggèrent que la Laponite réduit la mobilité des particules de sable par la formation d'un fluide poreux à propriétés solides. KEYWORDS: Nanoparticles, Liquefaction, Cyclic Triaxial Tests, Resonant Column Tests, Microstructure. 1 INTRODUCTION Liquefaction is a large-strain phenomenon occurring in saturated loose sand deposits during earthquakes causing large ground deformations, and significant damage to civil and industrial infrastructure (Ochoa-Cornejo et al. 2016; Youd et al. 2001). Liquefaction occurs as cyclic shear induces particle mobility; as undrained conditions prevail during earthquakes, excess pore water pressures generate, decreasing the effective stresses with each cycle of loading. Eventually, a transient condition of zero effective stress is reached, initiating liquefaction, large deformations, and ground failure (Castro 1975; Ishihara 1993). Liquefaction and its catastrophic damage were first observed in 1964, in the Alaska and Niigata Earthquakes, both of magnitude higher than Mw9.0 (Youd 1978), causing settlement and collapse of urban/industrial structures on saturated loose sand deposits. Liquefaction has also been observed in earthquakes such as El Maule 2010, Christchurch 2010, and Tohoku 2011 (Bhattacharya et al. 2011, Bradley 2012, Verdugo 2012). Liquefaction research initially focused on clean sands, and the factors controlling the behavior and resistance of sands against this phenomenon (Castro and Poulos 1977; Seed and Lee 1966; Seed et al. 1975). These studies highlighted the effect of the soil type, void ratio, initial effective confinement, overconsolidation ratio, and static shear on liquefaction. These studies concluded that liquefaction occurs mainly in sands; that for soils of similar nature, the higher the void ratio, the higher the liquefaction potential. Also, the higher the overconsolidation ratio, the higher the liquefaction resistance; and that it can manifest as either cyclic mobility or flow failure, depending on the initial static shear induced in the mass of soil. In the field, sand deposits are not clean. They have various percentages of fines of low-to-medium plasticity and even cementation. Studies on the effect of fine soils and cementation on liquefaction were popular in the 80’s and 90’s (Ishihara and Koseki 1989; Poulos et al. 1985; Troncoso and Verdugo 1985, Vaid and Sivathayalan 1996). Some authors suggested that the presence of fines reduces the liquefaction resistance (Troncoso and Verdugo 1985), while others suggested that liquefaction increases depending on the percentage of fines and plasticity (Koester 1994). Other studies found that plastic fines with PI > 10, as well as cementation, increase the liquefaction resistance (Clough et al. 1989; Ishihara and Koseki 1989). Since the early 2000’s, research efforts have aimed at developing methods to strengthen liquefaction resistance. The methods can be either active or passive. Active methods induce the densification of the soil with high energy sources, rearranging sand particles into a denser structure (vibro- compaction, dynamic compaction, and blast densification). Passive methods impact the soil behavior without affecting the fabric of the soil skeleton. They are more suitable in areas where civil infrastructure is close to, and around, the site of construction. For instance, a popular passive technique is the permeation grouting, which drills a bore, followed by the injection of a probe and the permeation of grouting material, without altering the fabric. However, this method can be time- consuming and expensive. The positive outcomes of liquefaction research during the last decades encouraged the development of new passive - 1071 - Proceedings of the 19th International Conference on Soil Mechanics and Geotechnical Engineering, Seoul 2017 methods to improve the soil right beneath constructed sites without disturbance. Examples of these techniques are the biocementation, colloidal silica grout, or the pore fluid engineering methods (El-Mohtar et al. 2013; Gallagher and Mitchell 2002; De Jong et al. 2006; Ochoa-Cornejo et al. 2016). Particularly, the pore fluid engineering method engineered a viscous thixotropic fluid in the void space of the sand skeleton with Bentonite solutions, increasing the liquefaction resistance. This increase was due to the extended elastic behavior of the granular soil induced by the high thixotropy of bentonite suspensions; in water, Bentonite forms a thixotropic fluid with a viscosity that increases with concentration and aging. The viscosity of bentonite suspensions is controlled to permeate it into the pore space between sand particles. As a result, the sand can withstand higher levels of shear strain. These findings were within the framework of an experimental program that considered the performance of cyclic triaxial and resonant column tests (El Mohtar et al. 2013). Bentonite is natural and safe, but complicated to implement in the field; Bentonite suspensions require chemical treatment to permeate it. The treatment aims at controlling the short-term viscosity and the rheological properties of the suspensions with sodium pyrophosphate (SPP). In addition, Bentonite suspensions might not be injectable in sands with fines. The experience with Bentonite encouraged to move forward by using Laponite, a synthetic nanoparticle. The use of Laponite skips/overcomes chemical treatment, particle gradation, and impurities. The clay-type particle Laponite is manufactured with a diameter ten times smaller than Bentonite, and it has a naturally delayed gelation period, allowing the permeation of larger soil volumes, respect to Bentonite. Also, Laponite has a higher plasticity index than Bentonite (1100 over 650), which would require smaller quantities of Laponite to strengthen the soil against liquefaction. 2 MATERIALS, EQUIPMENT, AND EXPERIMENTS 2.1. Materials This research used Ottawa Sand (C778) and Laponite RD. Ottawa sand is a clean uniform silica sand of fine-to-medium size. Its specific gravity, Gs, is 2.65, with emax=0.480, and emin=0.783 (Ochoa-Cornejo, 2015). Laponite RD is a synthetic nanoclay manufactured and commercialized by BYK Additives and Instruments. It is used as a rheology modifier in industries such as the cosmetics, inks, paints, and surface coatings. Single Laponite particles have a disk-like shape of ~25 nm in diameter and ~1 nm thickness. It is a 2:1 clay formed by a magnesium octahedral sheet sandwiched between two tetrahedral silica sheets (Figure 1(a)). Laponite has negative charges on both faces, and positive charges on the rim (Figure 1(b)). Its specific gravity is 2.57, and a plasticity index (PI) of ~1100% (El Howayek 2011), significantly greater than Bentonite (PI of ~350). In water, Laponite hydrates and swells, forming a monodisperse suspension. Laponite dispersions have early Newtonian behavior and delayed gelation process (Mongondry et al. 2005; Mourchid et al. 1995)). 2.2. Specimen Preparation and Testing Procedures Cyclic Triaxial and Resonant Column tests were conducted on dry pluviated specimens of clean sand, and sand with Laponite prepared at 1% of the nanomaterial by-dry-mass of sand. The skeleton relative densities of the specimens, Drsk, ranged between 15% and 25%, considering the emax and emin of the clean sand. The specimens were flushed with carbon dioxide (CO2) and de-ionized de-aired water, 200-300 kPa of backpressure, and B-values of 0.95. Specimens were isotropically consolidated to effective stresses of 100 kPa. Clean sand specimens were sheared after one hour of aging, while sand-Laponite specimens were aged for 72 hours. This period was found to be the time at which the developed rheological properties of Laponite pore fluid in the pore space reduced liquefaction susceptibility. In the case of triaxial testing, undrained cyclic loading was conducted with a vertical loading frequency of 1 Hz, and cyclic stress ratios that ranged between 0.1 and 0.25. For Resonant Colum tests, undrained cyclic loading was applied at resonance conditions. Resonant frequencies ranged between 50 Hz and 150 Hz. The angular uploads/Finance/ 2017-dynamic-behavior-of-sand-with-nanoparticles.pdf
Documents similaires
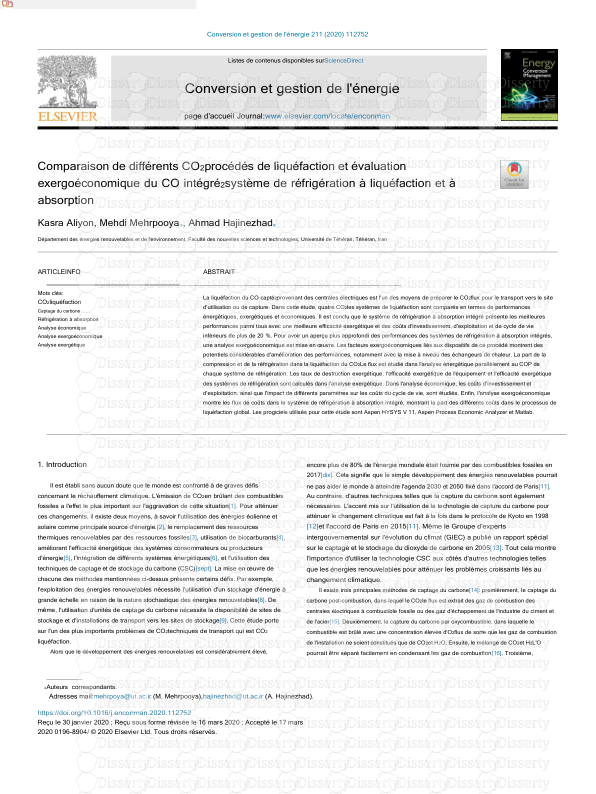
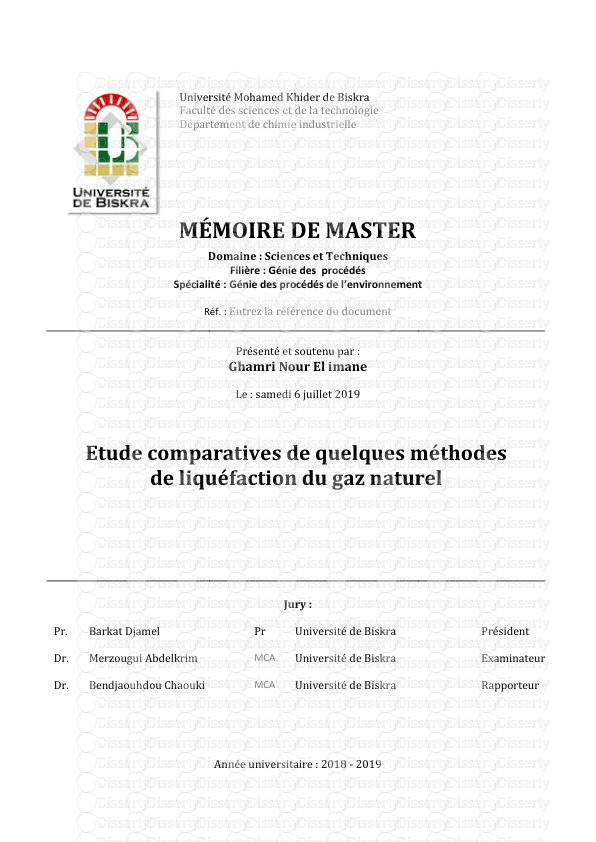
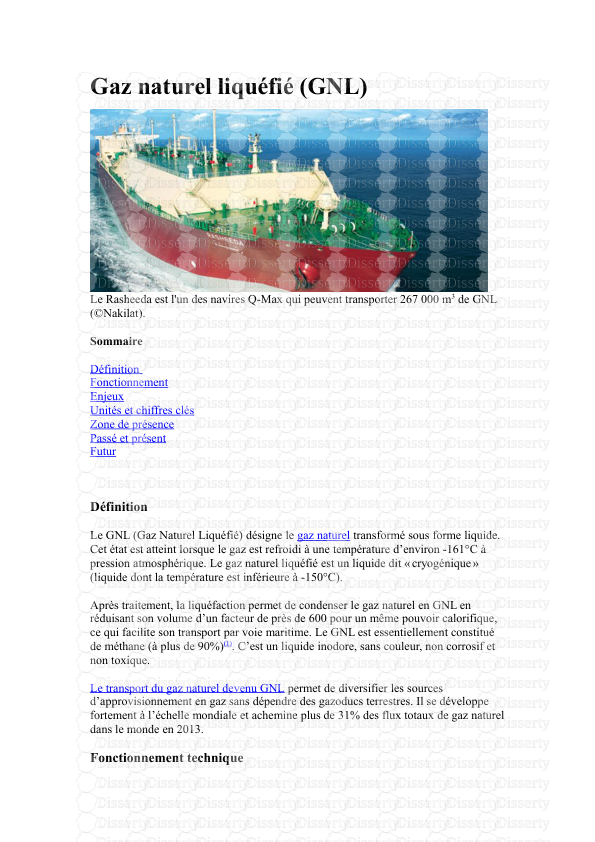
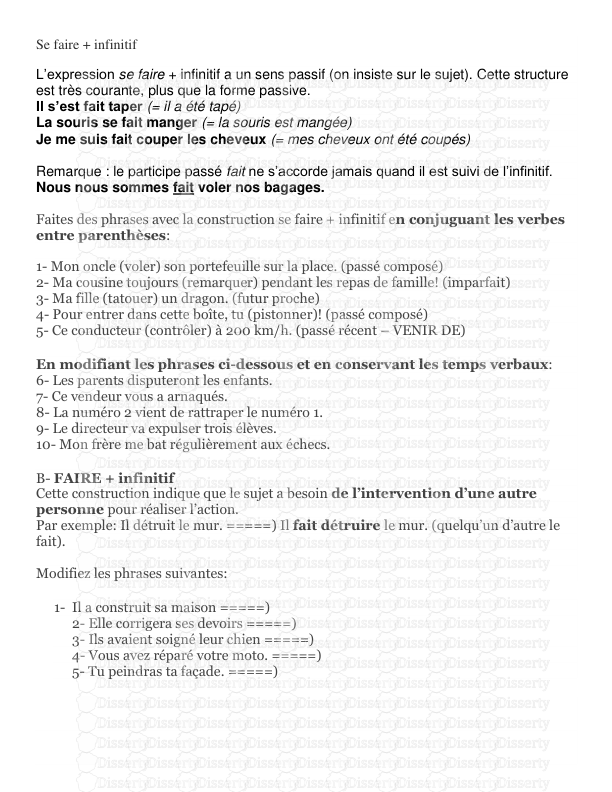
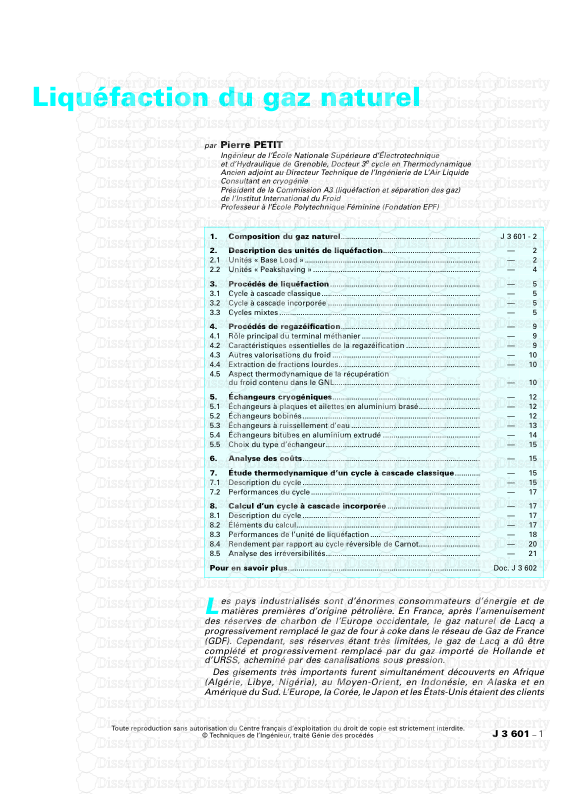
-
28
-
0
-
0
Licence et utilisation
Gratuit pour un usage personnel Attribution requise- Détails
- Publié le Mai 14, 2022
- Catégorie Business / Finance
- Langue French
- Taille du fichier 0.8968MB