ELECTRICAL IMPEDANCE SPECTROSCOPY Revision: 1.2 www.micronit.com www.micronit.c
ELECTRICAL IMPEDANCE SPECTROSCOPY Revision: 1.2 www.micronit.com www.micronit.com CONTENTS CONTENTS ................................................................................................................................................................ 2 INTRODUCTION ......................................................................................................................................................... 3 The design .................................................................................................................................................................... 3 EIS CAPABILITIES ....................................................................................................................................................... 5 EIS system set-up and testing ..................................................................................................................................... 6 Results of EIS measurements for sample beads ........................................................................................................ 7 Concluding remarks ..................................................................................................................................................... 9 References and acknowledgements......................................................................................................................... 10 Aside: Recommended cleaning technique ............................................................................................................... 10 CUSTOM EIS DESIGNS ............................................................................................................................................. 11 www.micronit.com INTRODUCTION The use of electrical impedance spectroscopy to detect and characterise cells can work as an effective in-line monitoring tool for microfluidic devices. There are several key advantages of this technique in the microfluidic environment including: • Fast throughput: ~1000 cells/s • Multiple parameter analysis • Integrates well with other analysis methods (i.e. optical detection) • Label free analysis method THE DESIGN All the Electrical Impedance Spectroscopy (EIS) chips are designed by Micronit and made of borosilicate glass and an inert interstitial layer of dry film resist for well-defined channels ideal for uniform flow through sensing region. These devices are delivered in a polymer (PP) cartridge of 75 x 25mm, which is compatible with the Fluidic Connect PRO chip holder. Our standard design for EIS-chips has a straight through channel 28µm deep and 30µm wide, making it suitable for complete blood count type applications. There are two sets of double electrodes, with planar separation of 20µm and 28µm depth separation as based on channel depth. A cross sectional schematic of the electrode layout can be seen in Figure 1. Figure 1: Cross sectional view of electrode layout www.micronit.com Do you want different features for your EIS-chips? • More inlets or outlets • Different channel dimension or electrode layouts • Extra functionalities or additional electrodes Contact us to have your own custom made EIS-chips. See page 11 for more information. www.micronit.com EIS CAPABILITIES Information, such as cell size, type discrimination and other quantitative characteristic information, can be measured for biological systems using impedance spectroscopy. EIS offers the advantage of acquiring this information from cells without the need to label them, as would be needed for characterisation using tools such as fluorescence microscopy. A standard cell can be considered as a membrane enclosing cytoplasm, which contains the nucleus of the cell as well as other subcellular components, a schematic of this can be seen to the left of Figure 2. All these different parts of the cell have different dielectric properties. For example, the cell membrane is generally not conductive, since it consists of a phospholipid bilayer with various channels formed by large proteins. In contrast, the cytoplasm of the cell typically contains a high concentration of ions, which makes it very conductive. Putting these things together, an electrical equivalent circuit model of the cell can be created, as shown to the right in Figure 2. Figure 2: Schematics of both a cell and a simplified circuit equivalent. Image courtesy of Zurich Instruments The electrical equivalent circuit model can be used to represent the various components such as the membrane as a capacitor and resistor in parallel and the cytoplasm as a single resistor. Finally, the surrounding environment can be included as both a capacitance and resistance in parallel. Then using impedance spectroscopy, the frequency response of the cell can be measured and fitted to this circuit model. This allows quantitative values, related to the different properties of the cell to be extracted, such as membrane thickness and cytoplasm conductivity. This information can even enable different cell types to be distinguished. Ultimately, the use of electrical impedance spectroscopy on the microfluidic scale are diverse and include applications such as: • Quality control within the Food industry (Wine, cheese and even potatoes) • Flow cytometry for counting and sorting of cells, biomarker detection and protein engineering • Blood analysis www.micronit.com EIS SYSTEM SET-UP AND TESTING In order to provide the best quality system for your investigations and characterisation of various particle types, we have joined forces with two innovative companies: Zurich Instruments AG and Fluigent SA. By utilising the Fluigent flow activation and control technology, the stable flow of particle suspensions through our EIS-chip is guaranteed. Whilst the use of Zurich Instruments’ high precision HF2IS Impedance Spectroscope provides a versatile measurement system of electrical signals for characterisation and monitoring. To highlight the capabilities of this co-developed system several validation experiments have been carried out. The Zurich Instrument HF2IS Impedance Spectroscope was connected to the Micronit EIS-chip through a purpose made connection kit FC_SPECTRO which consists of the Fluidic Connect Pro chip holder, inserts and electrical connection cables. The particle-laden fluids were delivered using the Fluigent MCFS-Flex system for accurate pressure flow control, see Figure 1: Cross sectional view of electrode layout Figure 3: for an image of the experimental set-up. The particles in these experiments consisted of 3µm and 5µm beads, where the concentration of 3µm beads was considerably higher than for the 5µm beads. Figure 3: Image of the electrical impedance spectroscopy experimental set-up www.micronit.com The detection is carried out using the double electrode set where the electric fields between the two electrode pairs allows a differential signal to be created by each passing particle, cell or bead as in the experiments discussed here. A schematic of how a particle in flow disturbs the electric field and creates a signal over time can be seen in Figure 4. Figure 4: Schematic of cell detection within an EIS-chip. Based on image courtesy of Zurich Instruments RESULTS OF EIS MEASUREMENTS FOR SAMPLE BEADS The initial results achieved using this set-up showed a signal was being received from the passing beads. A sinusoidal-like noise on the raw signal due to the mains current of the power supply was observed. This can be seen in the plots of the “Pre-processing” raw signal from each of the electrode pairs (X and Y), which are plotted to the left in Figure 5. Fortunately, this noise can be removed through post-processing. The acquired data was passed through a 50Hz software notch filter to remove the background electrical noise, which results in “Post- processing” data as shown to the right in Figure 5. Figure 5: Signal from the two electrode pairs without (pre-processing) and with (post-processing) 50Hz notch filtering www.micronit.com These notch filtered time series were then analysed to evaluate the detection of individual particles within the microchannel. The resulting information showed a clear signal was detected for both the 3µm and the 5µm beads, on both double electrode pairs. The signals from the 5µm beads display consistently larger amplitudes than the 3µm beads at both sensing areas, which can be seen in the data plotted in Figure 6. This is expected as the larger particles should give larger amplitude responses for set electric fields and separation of electrodes. Following the relationship of the measured amplitude of a bead being proportional to the radius cube of the bead. In this case we see, the 5µm beads gave roughly 75 and 120mV while the 3µm beads gave roughly 20 and 30mV response at positions X and Y respectively. These results both correspond well to the expected reduction of 4.6 times in detected amplitude. Figure 6: Signal of individual bead registered by the electrode pairs. On the left a signal for a 3m bead and on the right the signal for a 5m bead. To evaluate the consistency of detection across the whole cross section of the channel, a plot of velocity versus magnitude was created. The velocity for each bead can be calculated based on the delay between signal peaks from the electrode pairs. Theoretically, the magnitude of the peak voltage should be identical for the same sized beads, independent of the position within the channel. Thus, all identically sized beads should in theory have the same calculated velocity. However in these results, the beads were found not to flow exactly in the centre of the channel. Thus, the vertical position of the beads within the channel affected the results of the calculated velocity for two reasons. Firstly, the beads velocity is related to the velocity of the streamlines it is following, so closer to the walls the slower a bead flows. Secondly, the position of the bead in the channel disturbs the electrical fields differently and shifts the peak’s positions in time, thereby resulting in the spread of the calculated velocity for the same type of beads. www.micronit.com The resulting spread to the velocities acts as an effective indicator as to whether the cross sectional size of the channel is appropriate to the application. In terms of the results shown here, the channel is clearly better suited to the use of 5µm beads than 3µm beads as the spread to the velocities of the 3µm beads is larger. In this case the smaller spread for the results of the 5µm beads means the flow position is clearly more consistent, as can be seen in Figure 7. Figure 7: Peak voltage response of all beads compared to each velocity CONCLUDING REMARKS In general, the use of Micronit’s EIS-chip and connection system in conjunction with Fluigent’s MFCS-Flex flow control equipment and the Zurich Instruments’ uploads/s3/ eis-guide-v2.pdf
Documents similaires
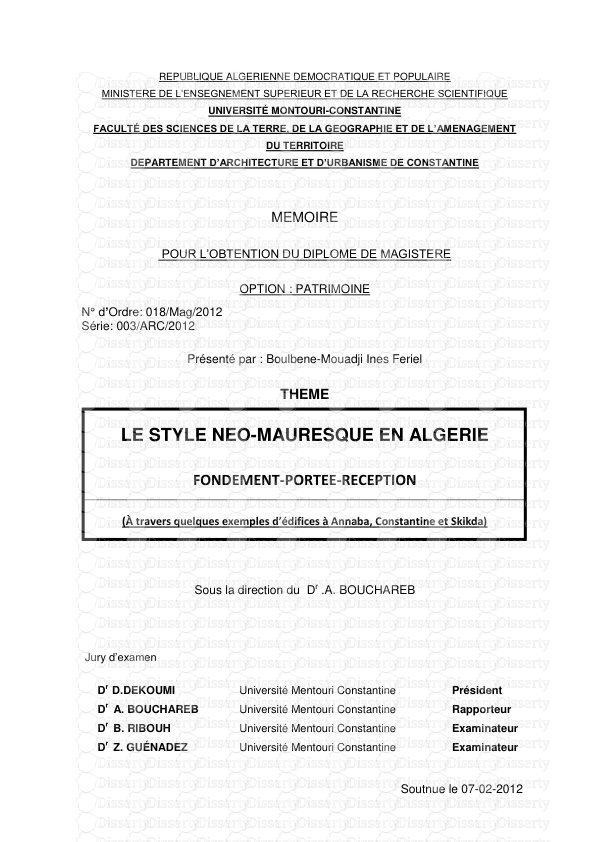
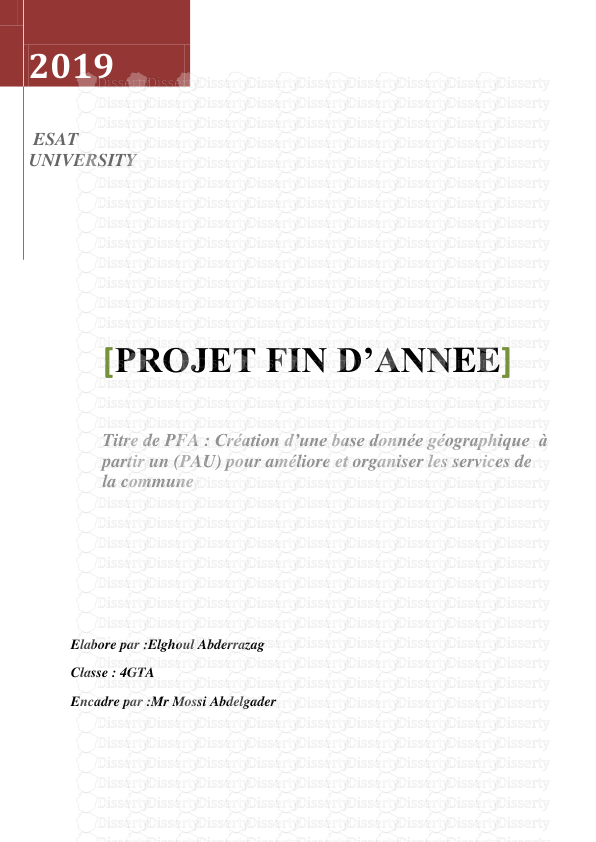
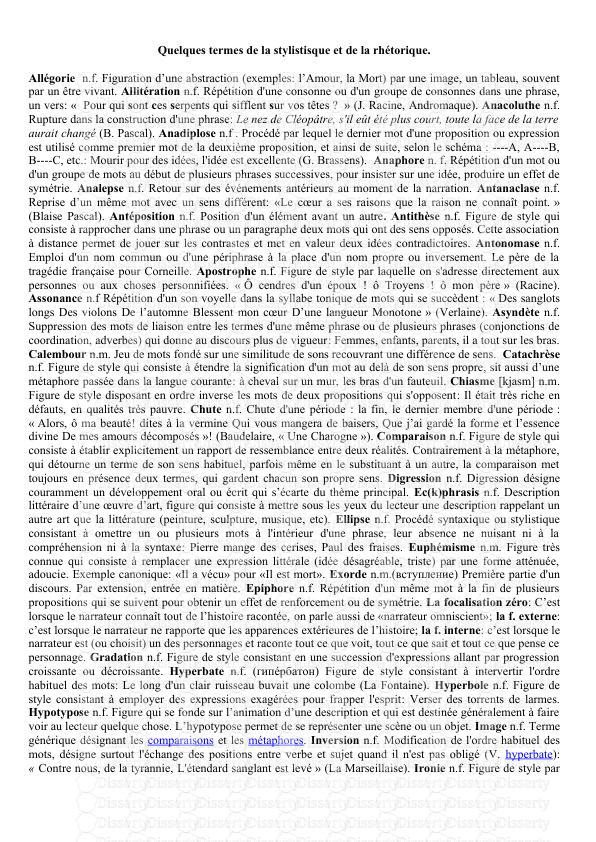
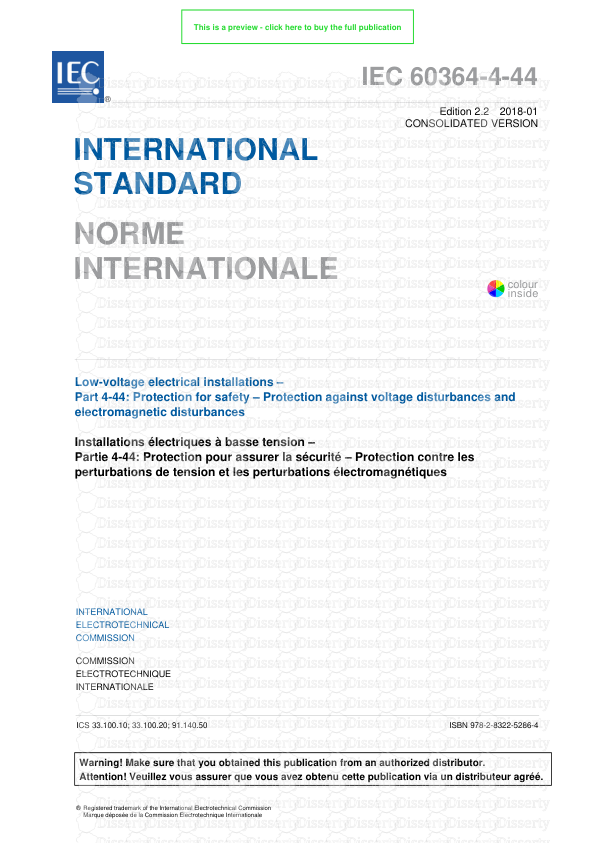
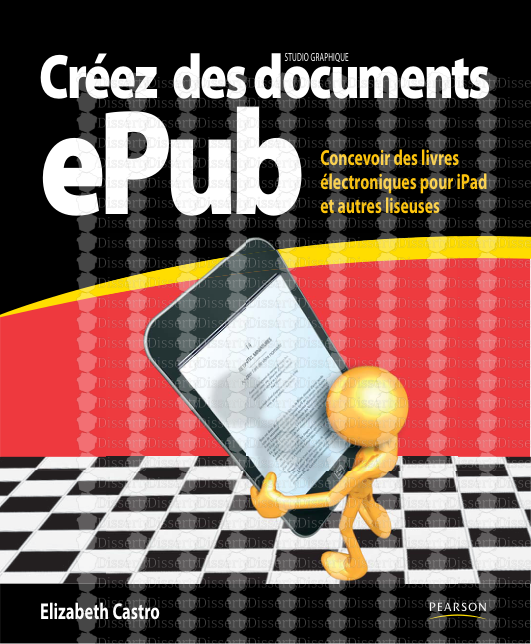
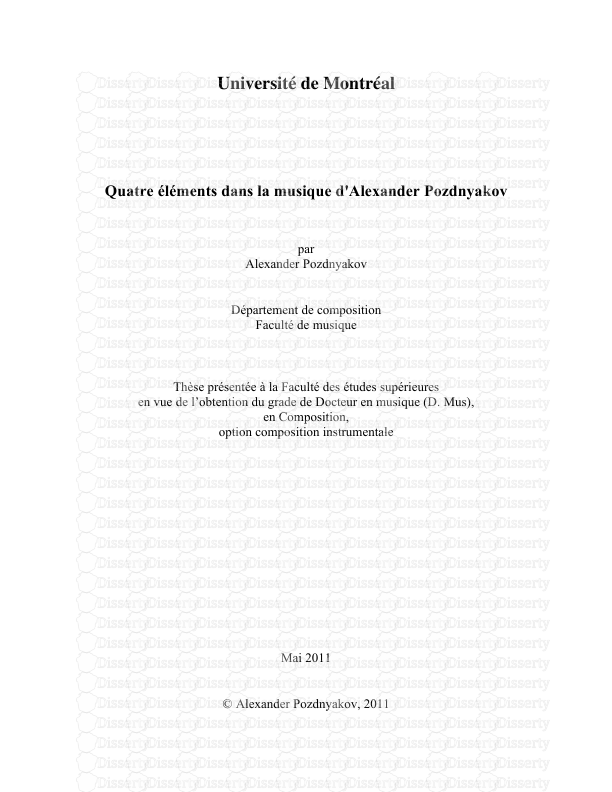
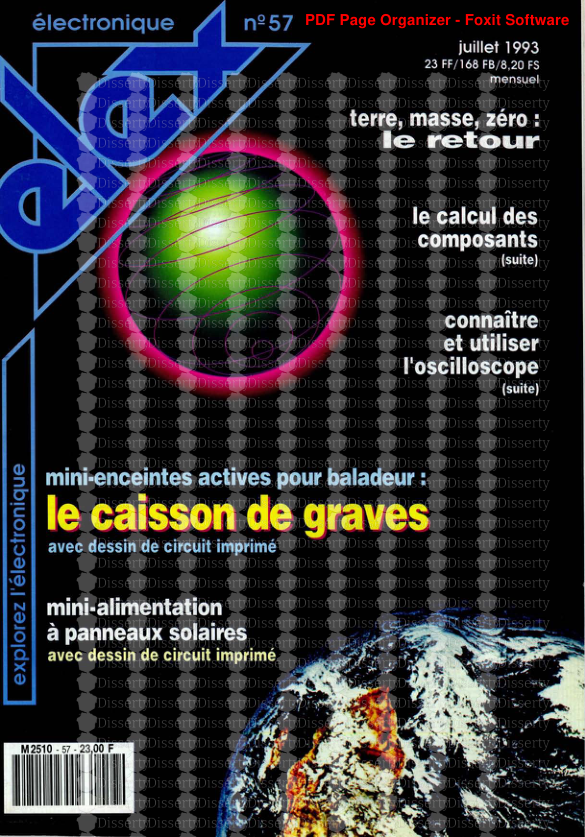
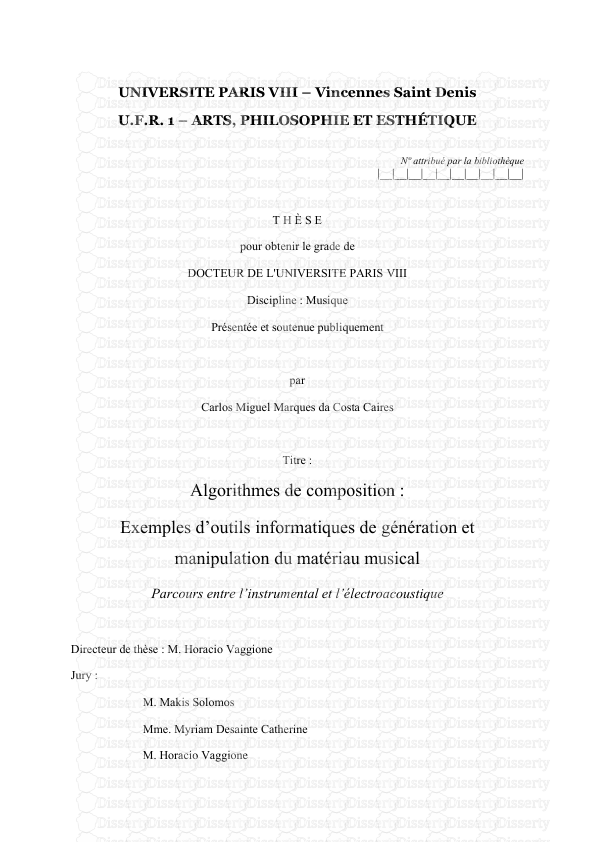
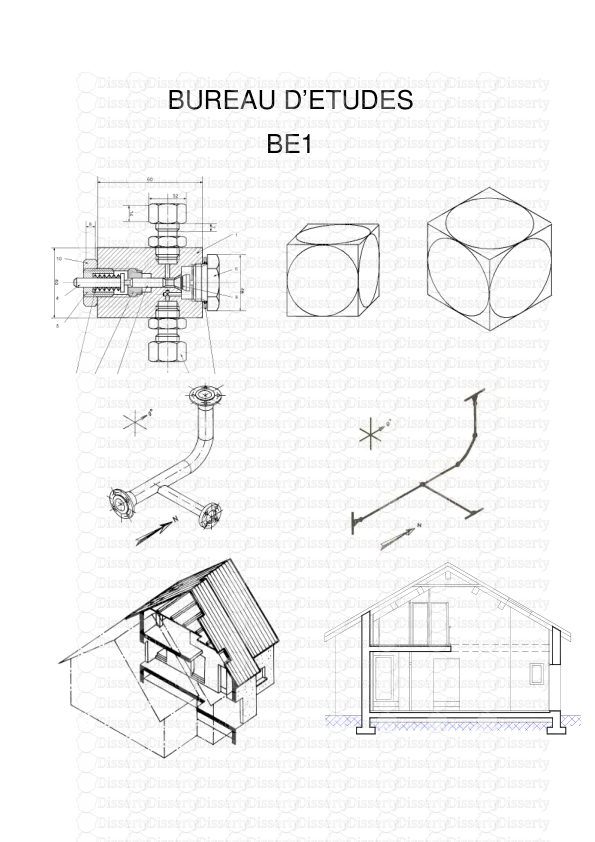
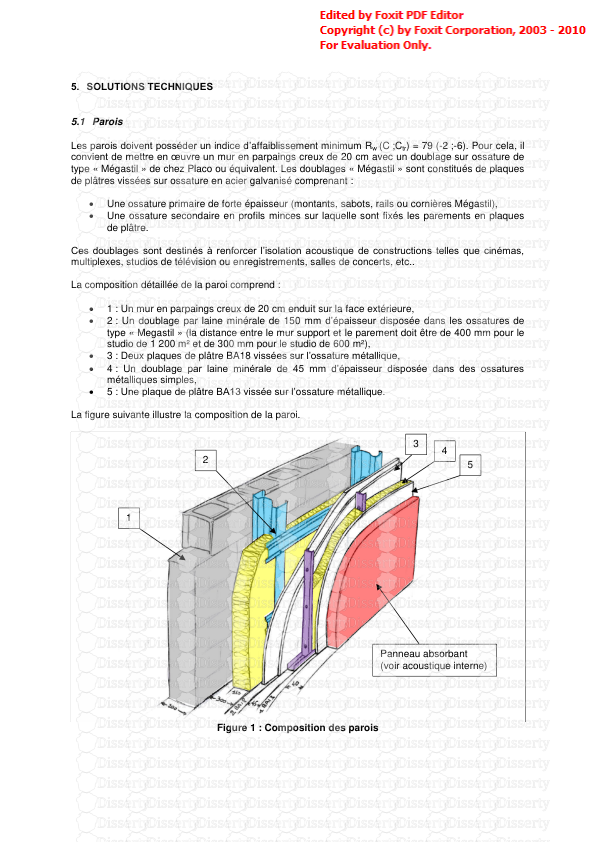
-
24
-
0
-
0
Licence et utilisation
Gratuit pour un usage personnel Attribution requise- Détails
- Publié le Jui 07, 2021
- Catégorie Creative Arts / Ar...
- Langue French
- Taille du fichier 1.2434MB