AXON GUIDE Chapter 5 ADVANCED METHODS IN ELECTROPHYSIOLOGY Recording from Xenop
AXON GUIDE Chapter 5 ADVANCED METHODS IN ELECTROPHYSIOLOGY Recording from Xenopus Oocytes The Xenopus oocyte expression system was introduced by Gurdon in 1971 as a means to study various aspects of the control of gene expression. Injection of exogenous DNA into the oocyte nucleus or mRNA into the cytoplasm led to the expression of functional proteins by the oocytes. Early studies dealt with the expression of proteins that are of little interest to electrophysiologists, such as globin, interferon and various viral proteins. Consequently, electrophysiologists and biophysicists paid scant attention to studies of protein expression in oocytes. However, beginning in 1982, Miledi and co-workers demonstrated that various types of ion channels and receptors could also be expressed in oocytes after injection of mRNAs that had been isolated from the appropriate tissue. For example, injection of Torpedo electric organ mRNA led to the expression of functional nicotinic acetylcholine receptors, while injection of rat brain mRNA resulted in the expression of a large number of different voltage- and ligand-gated ion channels, among them the voltage-gated Na+ channels, NMDA and non-NMDA subtypes of glutamate receptors, and GABAA receptors. Since these initial experiments, a number of workers have adopted this expression system to study various aspects of the structure and function of ion channels and receptors. Some of the more common types of studies that have employed expression of exogenous ion channels and receptors in oocytes include: (1) analyzing the properties of mutated channels as a means to understand structure-function relationships in ion channels; (2) studying the post-translational processing and the assembly of multisubunit channels and receptors; (3) comparing the properties of channels from various tissues expressed in a common environment; (4) examining the modulation of channel and receptor function by various second messenger systems; (5) analyzing various aspects of receptor-effector coupling; and (6) functional screening of cloned genes that encode channels and receptors (expression cloning). Most of these experiments involve electrophysiological recording from oocytes. This chapter describes the mechanics of recording from oocytes and points out some oocyte features of which one should be aware in order to obtain high-quality recordings. 92 / Chapter five What is a Xenopus Oocyte? Xenopus oocytes are egg precursors that, upon proper hormonal stimulation, pass through the frog's oviduct and become eggs, which can then be fertilized to make more frogs. Oocytes are stored in the abdominal cavity and can be removed surgically for experimental purposes. They go through six developmental stages (termed stages I to VI). Most researchers use only the large stage V and VI oocytes, which can be used interchangeably in electrophysiological experiments. Oocytes are found in clumps called ovarian lobes, which are made up of oocytes, connective tissue, blood vessels and follicle cells. Figure 5-1 shows an individual stage V or VI oocyte as found in an ovarian lobe. Follicle Cell Layer Animal Pole Vitelline Membrane Vegetal Pole Figure 5-1. Stage V or VI Oocytes as Found in an Ovarian Lobe The oocyte is a large cell, with a diameter of approximately 1 - 1.2 mm. It is surrounded by the vitelline membrane, which is a glycoprotein matrix that gives the oocyte some structural rigidity and helps it maintain a spherical shape. The mesh formed by this matrix is rather large; consequently, small molecules and even small proteins such as α-bungarotoxin (molecular weight 8,000 dalton) can interact with proteins on the oocyte surface. Since devitellinized oocytes are extremely fragile, the vitelline membrane is usually removed only for single-channel recording, where the surface of the oocyte must be sufficiently clean to allow the formation of a gigohm seal. A layer of follicle cells surrounds the vitelline membrane. The individual cells in the follicle cell layer are electrically coupled to each other and to the oocyte through gap junctions, so that electrical events taking place in the follicle cells can be detected in the oocyte. The follicle cell layer is a potential source for many problems. Therefore, most researchers remove it prior to mRNA injection or recording by one of two ways: (1) an extensive collagenase treatment to completely strip off the layer by immersing the oocyte for 1 - 3 hours in a calcium-free saline containing 2 mg/ml collagenase Type IA (Sigma Chemical Company, St. Louis, MO) until about half of the oocytes have been released from the ovarian lobe; or (2) a less extensive collagenase treatment followed by manual removal of the follicular layer with watchmaker's forceps. The latter is preferred, since oocytes that undergo the extensive collagenase treatment sometimes do not survive as long as those treated less extensively. Some of the problems introduced by the follicle cell layer are rather trivial. For instance, it is more difficult to impale a follicle-encased oocyte with an injection needle or a microelectrode. Other complications, however, can create major problems. The electrical coupling between the oocyte proper and the follicle cells means that one records from both. Numerous examples of oocyte Advanced Methods in Electrophysiology / 93 AXON GUIDE "endogenous" receptors that turned out to be in the follicle cell layer were published. In fact, the Xenopus oocyte is a nearly ideal expression system for ion channels and receptors since it has very few types of endogenous channels and receptors, an advantage that is defeated if the follicle cells are left on the oocyte. One of the striking features of a Xenopus oocyte is its two-toned color scheme: the animal pole hemisphere is dark colored, while the vegetal pole is light colored. This polarity is maintained inside the oocyte: the nucleus is found in the animal pole, and different populations of mRNAs exist in the hemispheres. In addition, a standing Cl- current flows from one pole to the other, indicating that the distribution of channels in the oocyte membrane is not homogeneous. Published reports showed that ACh receptors expressed from exogenous mRNAs are also unevenly distributed; although receptors were expressed all over the oocyte, more receptors were expressed on the vegetal pole. It is not clear if there is any relation between the site of RNA injection and the location of the expressed channels on the oocyte membrane. This non- homogeneity is not important for whole-cell recording, but could be important for single-channel recording. Two-Electrode Voltage Clamping of Oocytes Two factors need to be taken into consideration when voltage clamping oocytes. First, with an apparent surface area on the order of 106 µm2, there is an enormous amount of membrane that must be charged in order to clamp the cell. In fact, the situation is even worse than it may appear since there is considerable invagination of the surface membrane, thereby doubling or even tripling the surface area compared to an "ideal" spherical oocyte. Second, one can encounter currents up to 10 µA or larger after mRNA injection, potentially causing appreciable series resistance errors. In the case of currents from cloned K+ and Na+ channels, which activate rapidly and can give rise to very large currents, there may be an inadequate control of the voltage during the initial phases of channel activation. The response time (τ) of a voltage clamp to a step voltage change is, τ = R C A I m (1) where RI is the resistance of the current-passing electrode, Cm is the membrane capacitance, and A is the gain of the command amplifier. Since not much can be done about Cm (but see below), the only two things one can do in order to achieve fast clamping of the cell is to use the lowest RI and largest A possible. One "advantage" of the oocyte's large size is that one can use low- resistance electrodes for both the voltage-recording and current-passing electrodes. (This can hardly be regarded as an advantage, since the large size of the oocyte is what caused the problem in the first place). Electrodes that have resistances of 0.5 - 2 MΩ when filled with 3 M KCl are used. The particular type of glass is unimportant; both regular and fiber-filled electrode glass have been used successfully. Since the electrodes have rather large tip openings, they usually do not clog very often; the same set of electrodes can be used repeatedly during the course of a day. The electrodes' low resistance eliminates the need in a negative-capacitance circuit to correct for the frequency response of the voltage-recording electrode, thereby eliminating this potential source of clamp instability. To achieve the fastest response, the electrodes must be shielded in order to reduce capacitive coupling between them. Two rather simple ways of doing this are (1) placing a grounded sheet of metal between the two electrodes (making sure that it doesn't 94 / Chapter five make contact with the bath), or (2) wrapping a wire around a piece of tubing that fits over the voltage electrode like a sleeve, and then grounding the wire. In the latter case, the shield should extend as close to the bath as possible. The other parameter that one can control in order to maximize the speed of the voltage clamp is the command amplifier gain. As uploads/s3/ guide-ch-05.pdf
Documents similaires
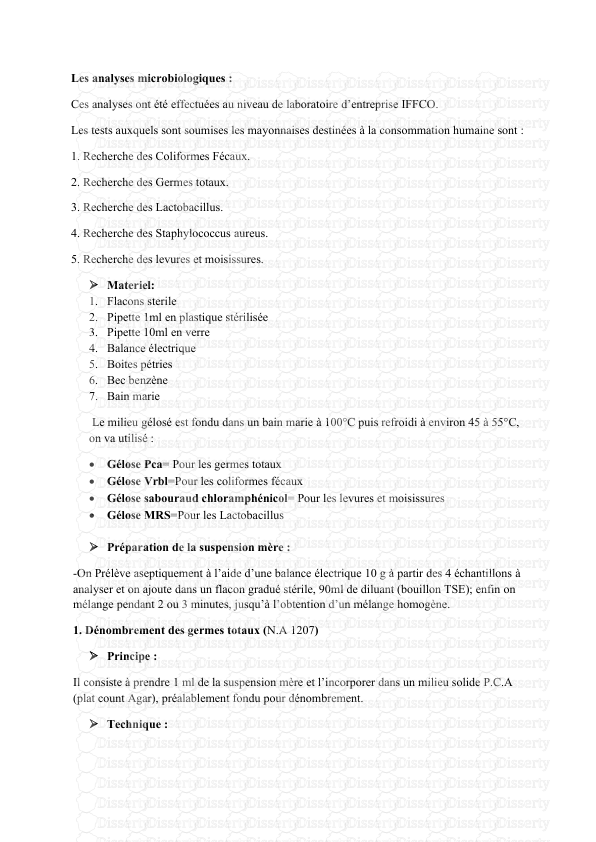
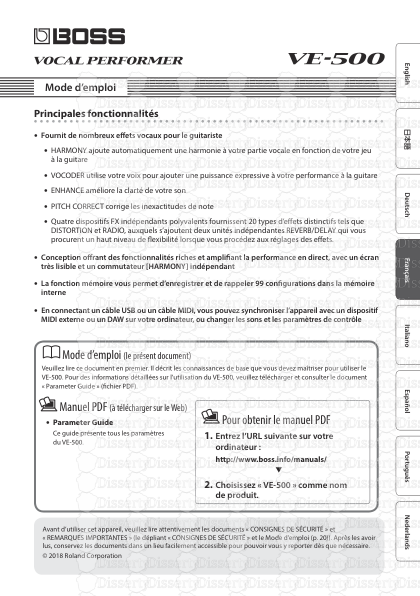
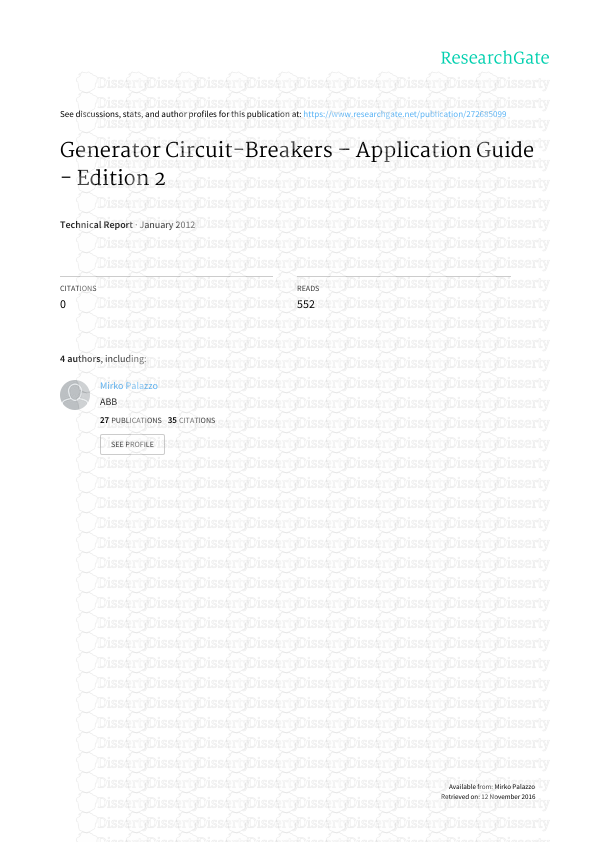
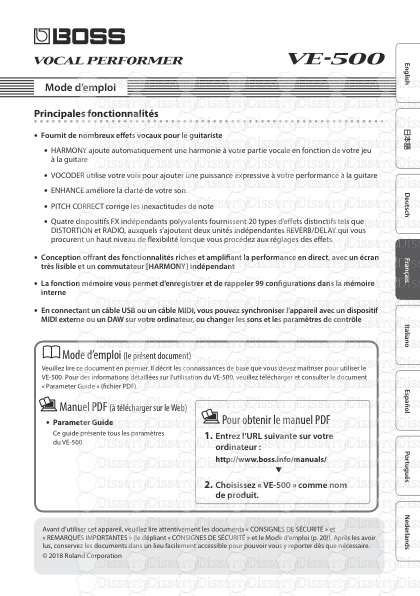
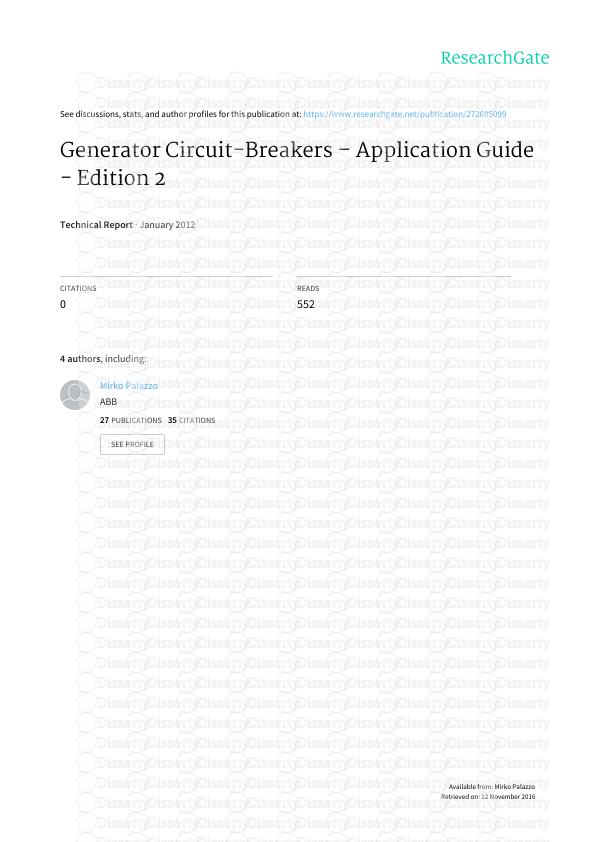
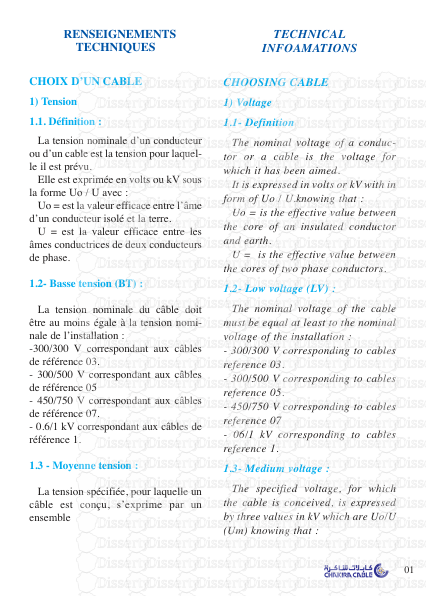
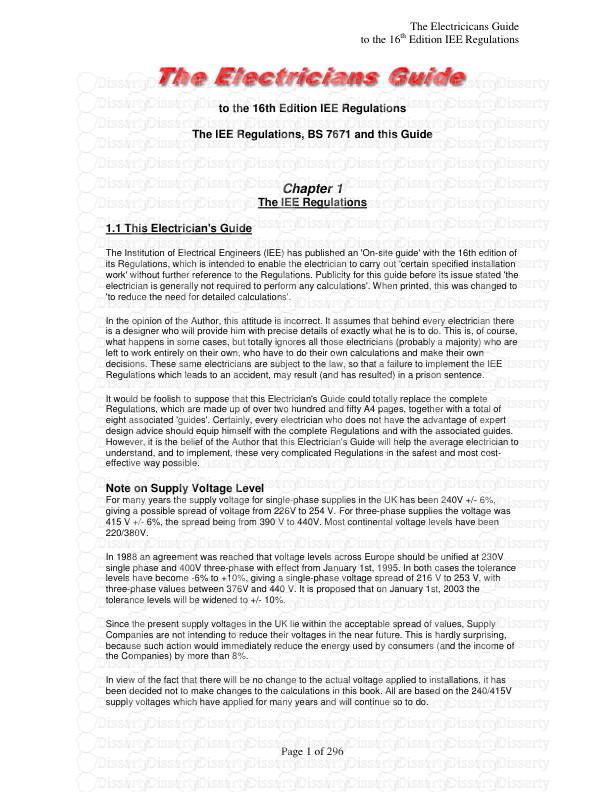
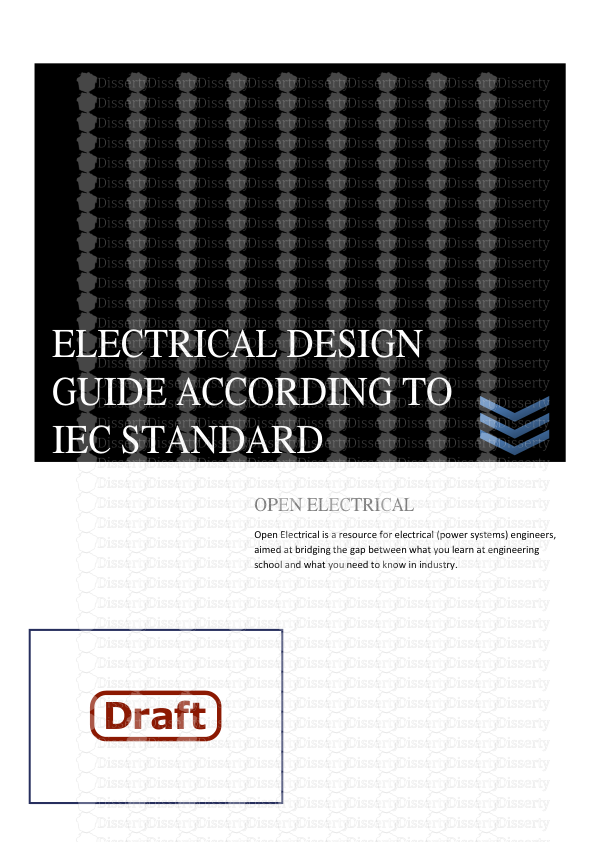
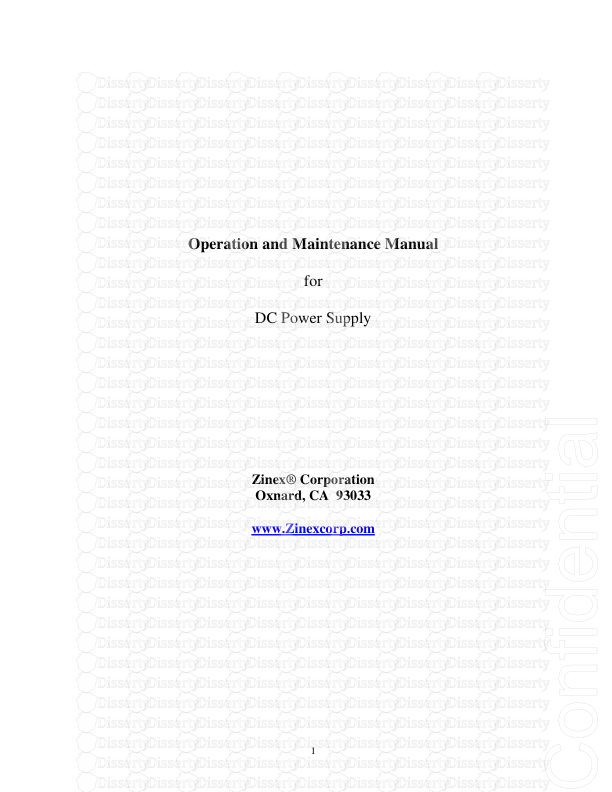
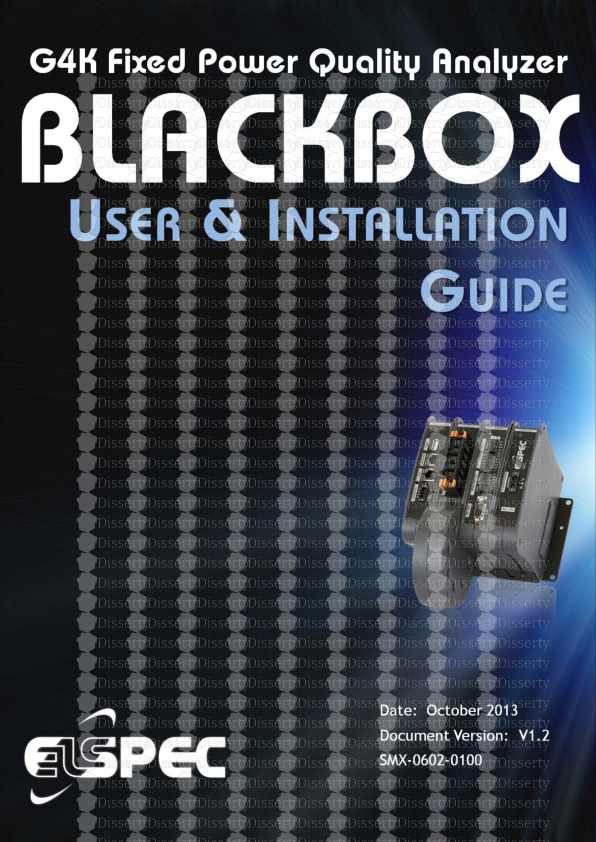
-
53
-
0
-
0
Licence et utilisation
Gratuit pour un usage personnel Attribution requise- Détails
- Publié le Aoû 18, 2021
- Catégorie Creative Arts / Ar...
- Langue French
- Taille du fichier 0.5440MB