Certified Flyers II BE76 Duchess Multi-Engine Study Guide 50 Airport Rd Suite 4
Certified Flyers II BE76 Duchess Multi-Engine Study Guide 50 Airport Rd Suite 40 Morristown, NJ 07960 Tel: (973) 539-4080 Fax: (973) 539-1836 www.certifiedflyers.com April 2012 Certified Flyers II Duchess Multi-Engine Study Guide 1 The Certified Flyers Multi-Engine Study Guide is for reference only and is intended only to supplement, not replace, manufacturer and FAA publications such as the pilots operating handbook. All pilots must operate the aircraft in accordance with the Pilot’s Operating Handbook and abide by Federal Aviation Regulations. Contents SECTION 1: MULTI-ENGINE AERODYNAMICS………………………………………….2 SECTION 2: AIRCRAFT SYSTEMS AND LIMITATIONS………………………………...7 SECTION 3: NORMAL FLIGHT PROCEDURES………………………………………….15 SECTION 4: EMERGENCY PROCEDURES……………………………………………….22 SECTION 5: MULTI-ENGINE IN-FLIGHT MANEUVERS………………………………27 APPENDIX 1: BE76 DUCHESS QUICK REFERENCE CHECKLIST…………………...31 APPENDIX 2: INSTRUMENT APPROACH BRIEFING “5 PHASES”…………………..33 Certified Flyers II Duchess Multi-Engine Study Guide 2 Section 1: Engine-out Aerodynamics TURNING TENDENCIES The turning tendencies that affect a single engine aircraft (p-factor, torque, spiraling slipstream, gyroscopic precession) will also affect a multi-engine aircraft. Because a multi-engine aircraft has two engines many of these turning tendencies increase. A twin engine aircraft where both engines are rotating the same direction is called a conventional twin. To combat p-factor and torque, aircraft with counter-rotating propellers have been developed (the BE76 Duchess has counter-rotating propellers). The p-factor and torque from counter-rotating propellers cancel each other out which results in less rudder needed to oppose their turning tendencies. CRITICAL ENGINE A critical engine is the engine which, if lost, will most adversely affect the performance and handling characteristics of the aircraft. The affect of the critical engine is most significant when the aircraft is operating at low airspeed with a high power setting (thus more p-factor and torque). On a conventional twin with propellers rotating clockwise, the critical engine is the left engine. On an aircraft such as the BE76 Duchess with counter-rotating propellers there is not a critical engine because the yawing and rolling caused from losing either engine is identical. There are four factors which determine if an engine is critical: 1. P-Factor 2. Accelerated Slipstream 3. Spiraling Slipstream 4. Torque WHAT HAPPENS WHEN AN ENGINE FAILS? Two things happen when an engine fails: Yaw and Roll towards the dead engine because now lift, thrust, and drag act on your aircraft asymmetrically. 1. Yaw- Asymmetrical thrust will cause a yawing motion around the C.G. toward the inoperative engine. 2. Roll- Induced flow (Accelerated Slipstream- extra lift created by accelerated air over the wing) from the operating engine and lack of induced flow from the inoperative engine causes asymmetric lift on wings. This results in a rolling moment around the C.G. towards the inoperative engine. 3. Roll- Yawing moment from the asymmetric thrust will cause the operating engine to move faster through the air as the aircraft yaws. This causes faster velocity air to flow Certified Flyers II Duchess Multi-Engine Study Guide 3 over the operating engines wing causing more lift on that wing, thus roll towards the inoperative engine. To counteract this roll and yaw, rudder pressure must be applied to the side of the operational engine to oppose these forces. Hence, “Dead foot- Dead engine”. ZERO SIDESLIP CONDITION The solution to maintaining aircraft heading and reducing drag is to improve performance using the Zero Sideslip Condition. When the aircraft is banked into the operating engine (2-5 degrees of bank), the dihedral of the wing will create a horizontal component of lift. The horizontal component of lift minimizes rudder deflection required to align the longitudinal axis of the aircraft to the relative wind. In addition to banking into the operating engine, the appropriate amount of rudder required is indicated by the inclinometer ball being “split” towards the operating engine’s side. The zero sideslip condition must be flown for optimum aircraft performance. CLIMB PERFORMANCE AND SERVICE CEILING Climb performance is dependent on the excess power needed to overcome drag. When a twin-engine airplane loses an engine, the airplane loses 50% of its available power. This power loss results in a loss of approximately 80% of the aircraft’s excess power and climb performance. Drag is a major factor relative to the amount of excess power available. An increase in drag (such as the loss of one engine) must be offset by additional power. This additional power is now taken from the excess power, making it unavailable to aid the aircraft in the climb. When an engine is lost, maximize thrust (full power) and minimize drag (flaps and gear up, feather prop, etc) in order to achieve optimum single engine climb performance. Drag Factors: 1. Full Flaps- ~400 fpm approx. 2. Windmilling Prop- ~400 fpm approx. 3. Gear Extended- ~150 fpm approx. Single-engine service ceiling- the highest altitude at which the airplane can maintain a steady rate of climb of 50 fpm with one engine operating at full power and one engine’s propeller feathered Certified Flyers II Duchess Multi-Engine Study Guide 4 Single-engine absolute ceiling- the altitude where climb is no longer possible with one engine operating at full power and one engine’s propeller is feathered Vmc- MINIMUM CONTROL SPEED Rudder is applied to counteract yaw and roll from an inoperative engine in a multi-engine aircraft. As airspeed decreases the rudder becomes less effective, eventually an airspeed will be reached where full rudder deflection is required to maintain directional control. At this point, any further airspeed reduction will result in a loss of directional control. This airspeed is Vmc, the airspeed at which it is still possible to maintain directional control with an engine inoperative. §23.149 Minimum Control Speed Vmc is the calibrated airspeed, at which, when the critical engine is suddenly made inoperative it is possible to: 1. Maintain control of the airplane with the engine still inoperative 2. Maintain straight flight at the same speed with an angle of bank not more than 5 degrees. The method used to simulate critical engine failure must represent the most critical mode of powerplant failure expected in service with respect to controllability. Vmc must not exceed 1.2 Vs1 at maximum takeoff weight. Vmc must be determined with: 1. Most unfavorable weight (not necessarily gross weight) 2. Most unfavorable center of gravity position 3. The airplane airborne and the ground effect negligible 4. Maximum available takeoff power initially on each engine 5. The airplane trimmed for takeoff 6. Flaps in the takeoff position 7. Landing gear retracted 8. All propeller controls in the recommended takeoff position When recovering from Vmc: 1. The rudder pedal force required to maintain control must not exceed 150 pounds 2. It must not be necessary to reduce power of the operative engine(s) 3. The airplane must not assume any dangerous attitude 4. It must be possible to prevent a heading change of more than 20 degrees RECOGNIZING AND RECOVERING FROM Vmc There are four warning signs that Vmc is occurring or about to occur: 1. Loss of directional control- the rudder pedal is depressed to its fullest travel and the airplane is still turning towards the inoperative engine Certified Flyers II Duchess Multi-Engine Study Guide 5 2. Stall warning horn- a single engine stall may be just as dangerous as running out of rudder authority and could even result in a spin 3. Buffeting before the stall- same reason as the stall warning horn 4. A rapid decay of control effectiveness- any loss of control effectiveness could result in a loss of control of the aircraft To recover from Vmc, these two actions must occur simultaneously: 1. Reduce power on the operating engine- this will reduce the asymmetrical thrust causing the Vmc in the first place (remember, reducing power all the way to idle may help stop the Vmc, but the loss of airspeed and power can lead to a stall) 2. Pitch down- lowering the nose of the airplane will increase the forward airspeed making the rudder more effective in regaining and maintaining directional control AND IT’S RELATIONSHIP TO STALL SPEED As density altitude increases, Vmc speed will decrease because as density altitude increases engine power will decrease (less engine power at higher density altitude less asymmetric thrus less yaw towards dead engine= lower ). Stall speed is an indicated airspeed which will remain constant as altitude increases or decreases. FACTORS AFFECTING Vmc AND SINGLE ENGINE PERFORMANCE Vmc is defined using a very specific set of conditions, thus published Vmc and actual Vmc can be two very different numbers. Remember, Vmc only addresses directional control and is not related to aircraft performance. While controllability is important, the degradation of performance in a single engine situation also has serious consequences. A variety of factors affect both controllability and performance with one engine inoperative, such as aircraft configuration, flight conditions, and pilot action. In some cases, an element which provides an increase in controllability (translating into a decrease in Vmc) may actually hinder performance. Refer to the chart on the next page to review how certain factors affect both Vmc and performance. Certified Flyers II Duchess Multi-Engine Study Guide 6 EFFECT ON Vmc PERFORMANCE Power Increase Up- more yaw Up- more power Temp uploads/Management/ multi-study-guide.pdf
Documents similaires
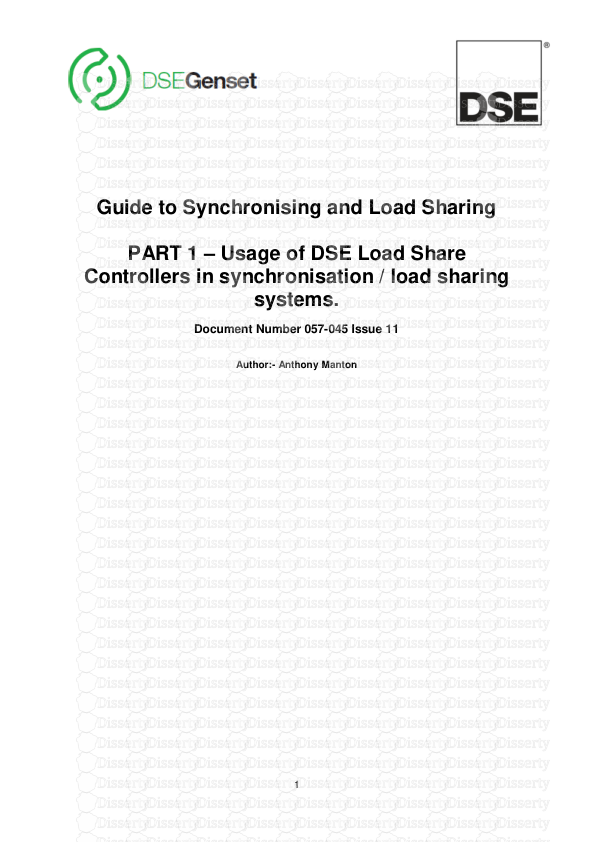
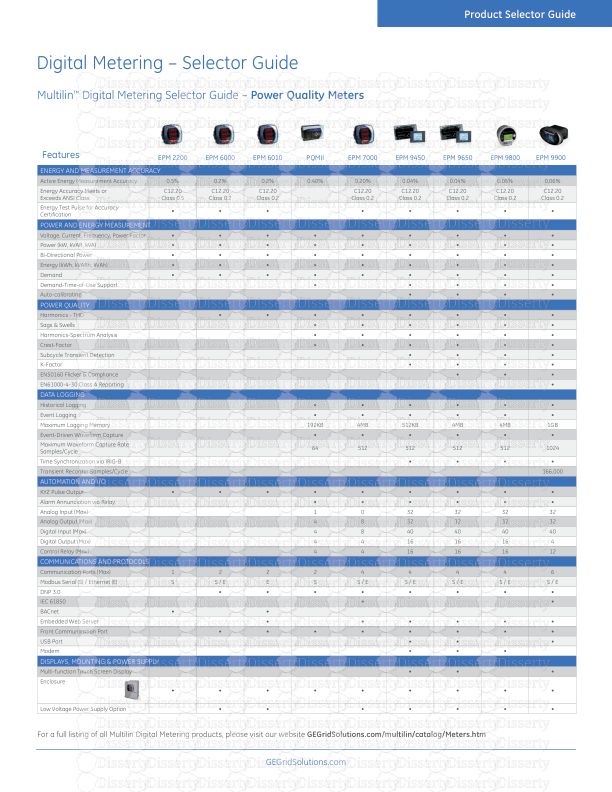
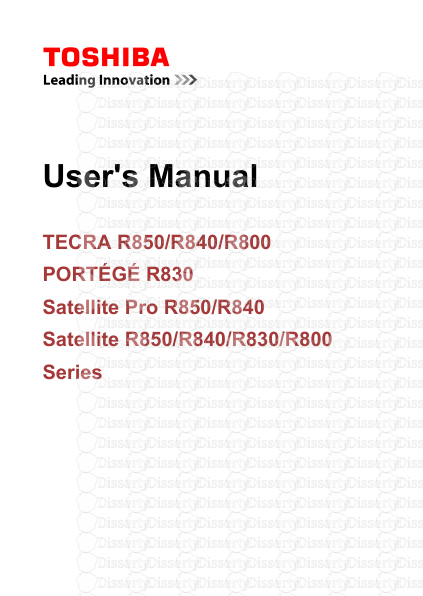
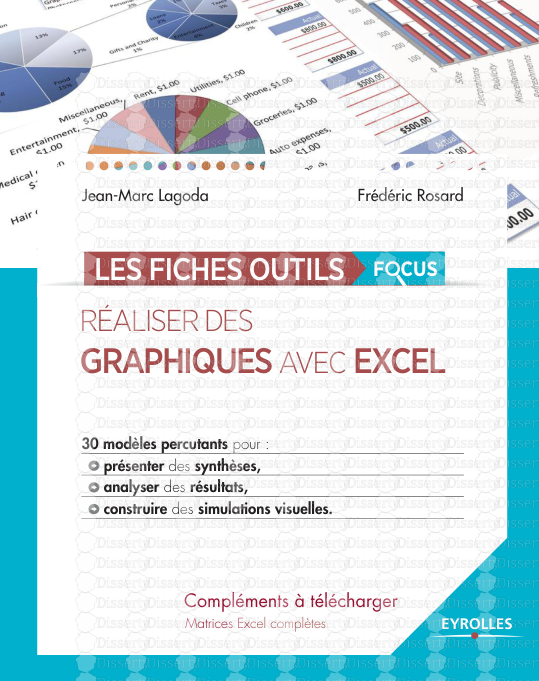
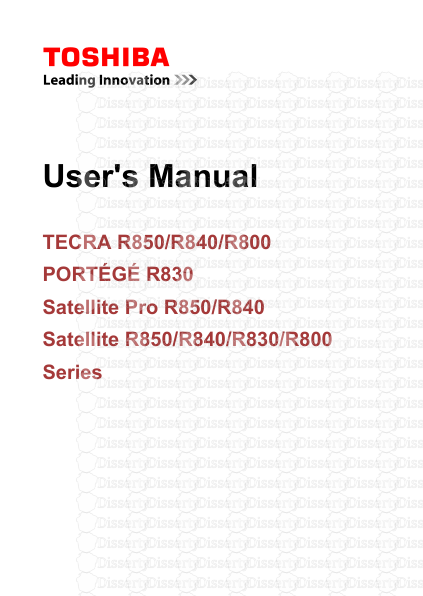
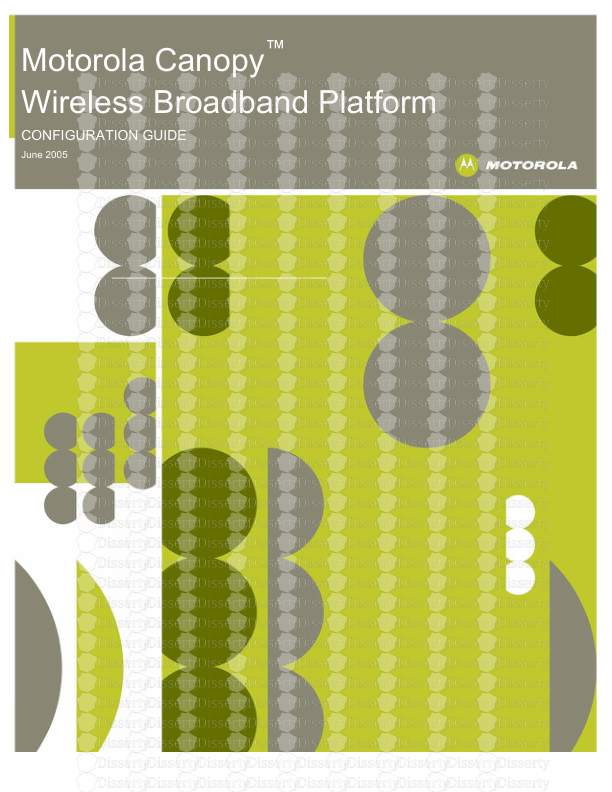
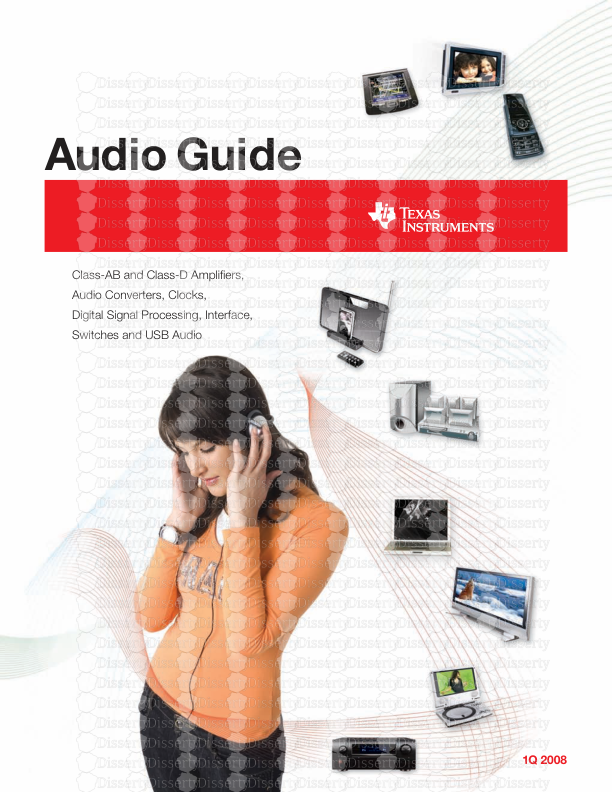
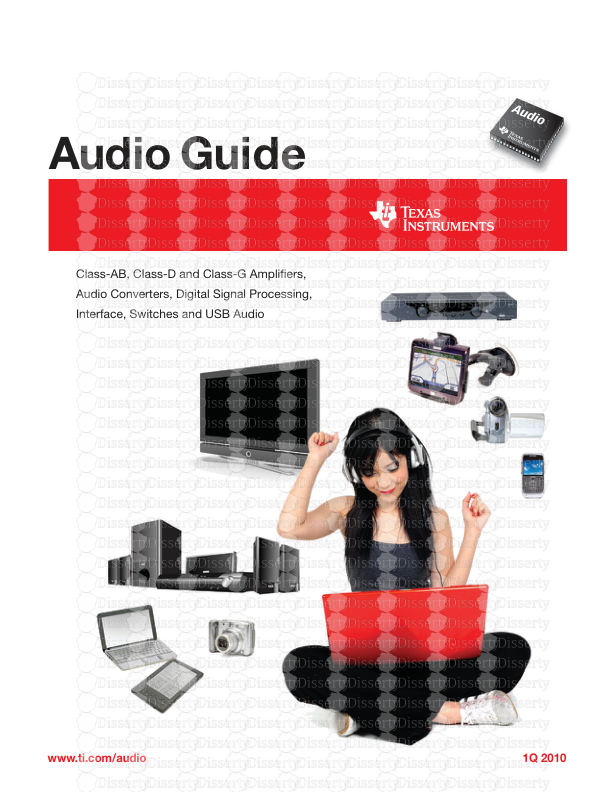
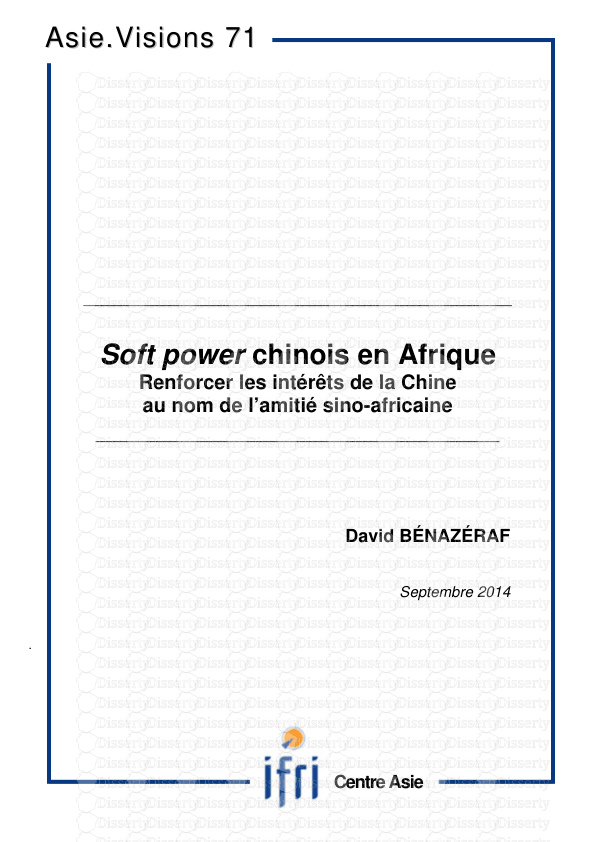
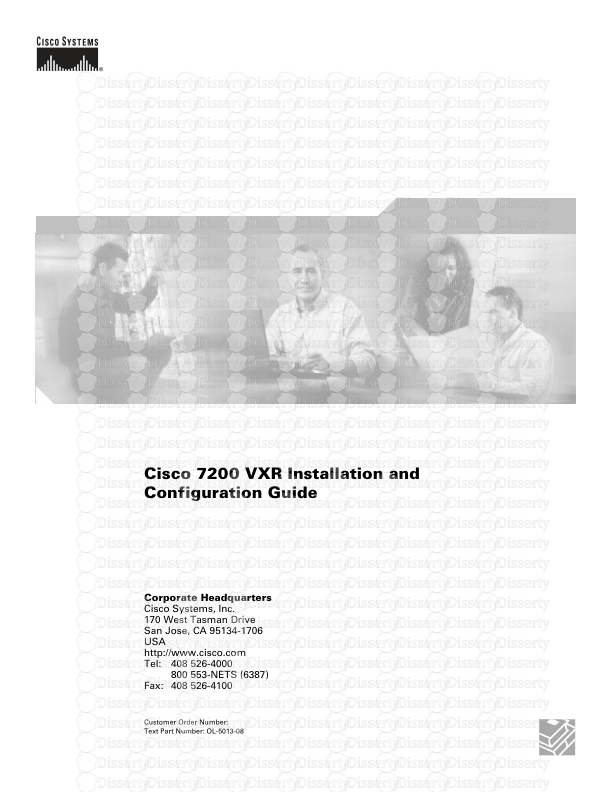
-
10
-
0
-
0
Licence et utilisation
Gratuit pour un usage personnel Attribution requise- Détails
- Publié le Jul 02, 2022
- Catégorie Management
- Langue French
- Taille du fichier 1.9519MB